UT Research on Methane Hydrate Could Transform the Energy Landscape

The middle of the Gulf of Mexico is a busier place than you might think. Its deep, blue waters are dotted with passing boats and energy platforms. Last summer, the Q4000 was one of them.
Most of the time, the Q4000 is an industry rig. It has two remotely operated vehicles for inspecting and servicing wellheads and observing boreholes on the ocean floor. A wall of shiny gold plaques in the vessel’s common room commemorates the rig’s role in capping the Deepwater Horizon oil spill.
But last year The University of Texas at Austin outfitted the Q4000 to new ends for a special mission. The rig was turned into a scientific drilling vessel, one that could take core samples of methane hydrate—a super-compressed form of gas trapped inside a cage of water ice, and one of the largest caches of carbon on the planet.
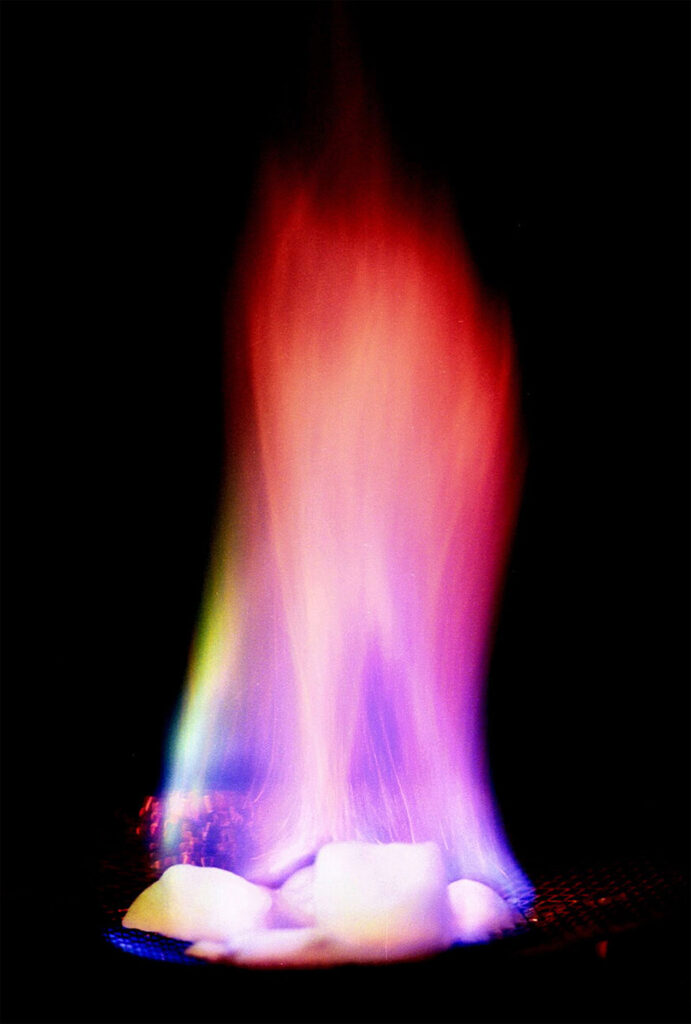
Walker Ridge, the target for the UT-led mission, is just one of many methane hydrate reservoirs under the seafloor of the Gulf and around the world. Layers of the mysterious substance are also found beneath ice sheets and Arctic permafrost. Scientists estimate that, globally, the supply of hydrates may hold anywhere from 5 percent to 22 percent of the Earth’s mobile carbon—the carbon that plays the most immediate role in shaping climate, environment, and life on Earth. For comparison, that is up to 10 times as much carbon as is in the atmosphere.
There are big questions about how methane hydrate may shape the energy landscape, with undersea reservoirs potentially holding an untapped supply of natural gas, particularly for energy-poor countries. The solid hydrate is incredibly energy-dense, with each unit of methane hydrate having 165 times the energy of an equivalent volume of gas at surface conditions.
But methane is also a potent greenhouse gas, with about 25 times the heat-trapping potential of carbon dioxide. There are big questions here, too, about what conditions may lead the methane to escape from its hydrate cage and seep into the wider world—and the impacts this could have on the environment and climate.
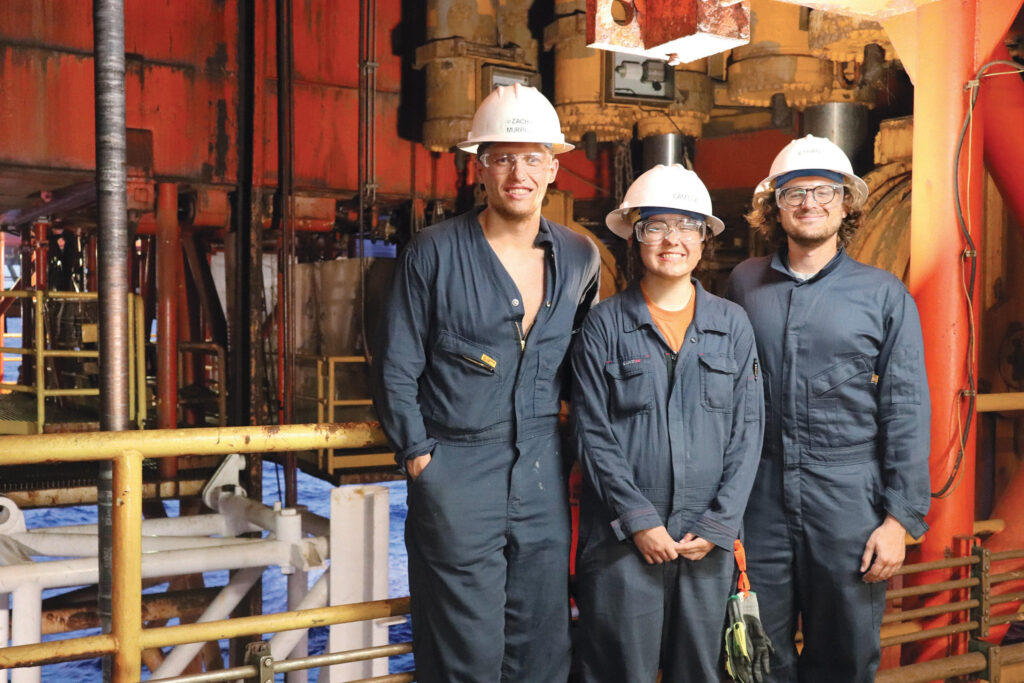
Answering those questions requires getting an up-close look at a methane hydrate reservoir from top to bottom. That’s exactly what Jackson School of Geosciences Professor Peter Flemings, the chief scientist of the mission, set out to do.
“One of the amazing things about The University of Texas is that if you say you want to do something really big, they will help you find a way to make it happen,” Flemings says. “There’s no bigger example I can think of than this.”
Joining Flemings offshore was a team of scientific collaborators from four other universities, the U.S. Geological Survey (USGS), and the technical company Geotek Coring. That’s not to mention the 108 additional crew members—the cooks, cleaners, drillers, and more—providing support so the mission could run 24 hours a day for a month straight. And offshore rigs don’t come cheap; it costs half a million dollars a day to keep the Q4000 running. The mission was made possible by a grant of more than $100 million from the U.S. Department of Energy, one of the largest ever awarded to a university. It reflects the importance of methane hydrate—and how much there is to discover about the mysterious, energy-rich substance at the bottom of the sea.
The mission wasn’t the first time Flemings set off to sea aboard the Q4000. In 2017, he led a mission on the vessel that extracted the first pressure cores of methane hydrate ever recovered from the Gulf of Mexico.
It’s impossible to keep methane hydrate intact unless it’s kept under pressure. If the pressure lessens, the methane escapes, bubbling away. While the 13 pressure cores recovered during the mission provided valuable scientific samples, the key accomplishment was a technological one: The scientists proved that the pressure-coring tool developed to keep the samples intact worked.
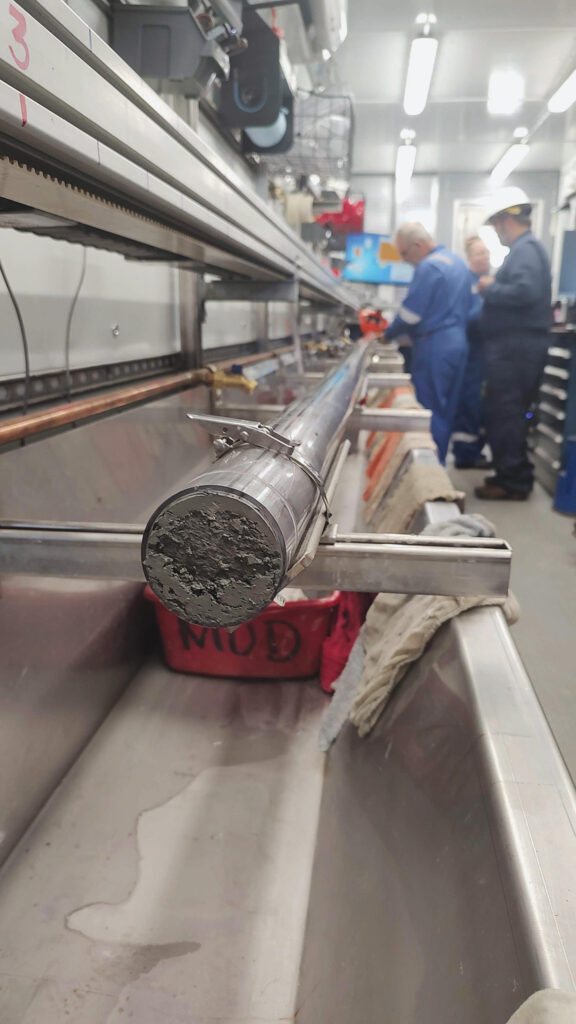
This success opened the door to a bigger science mission—one that could sample an entire methane hydrate reservoir instead of just a single layer within it. Flemings and the science team set their sights on taking cores—both pressurized and conventional—that spanned from the start of the seafloor to more than 3,000 feet below it.
“UT-GOM2-1 was a technology test,” Flemings says, referring to the first mission. “The true science, in terms of a systems understanding of the reservoir, will come from UT-GOM2-2.”
The 24-member, offshore science team for last summer’s mission included a wide range of perspectives, from sedimentologists to microbiologists and geochemists. The team also included graduate students, postdoctoral researchers, and a sole undergraduate researcher, Camila Van Der Maal.
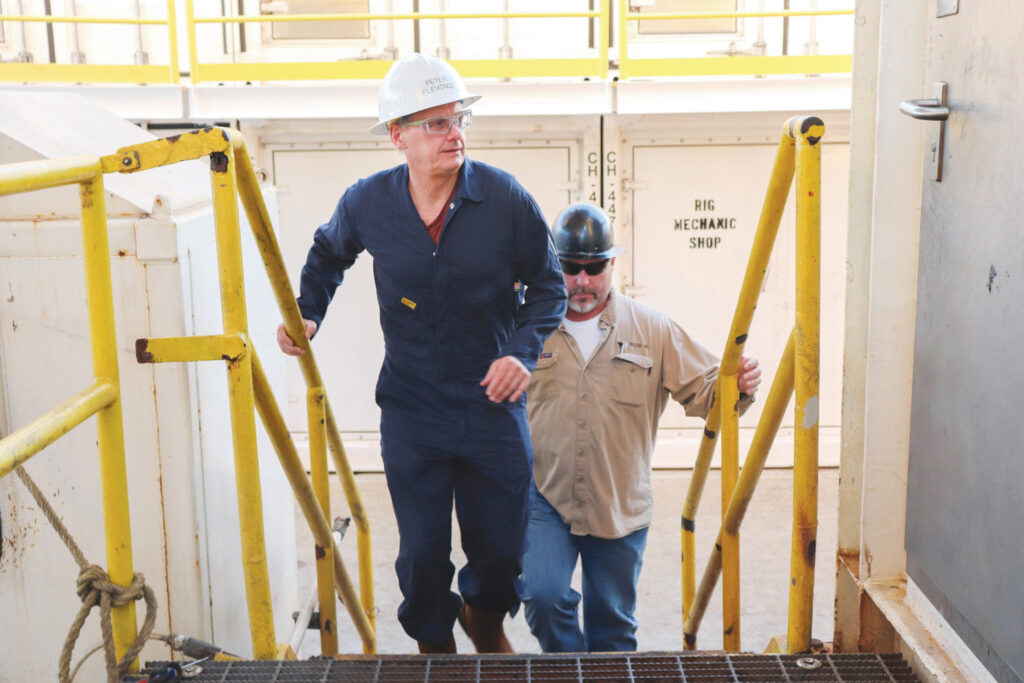
At the time of the mission, Van Der Maal was a first-year geophysics student at the Jackson School who had only spent a few months working in Flemings’ core lab, degassing pressure cores from the 2017 mission. On the summer mission she worked with Rick Colwell, a microbiologist and professor at Oregon State University. Microbiology was new to her, but that could be said about much of the mission. She had never traveled to another state in the U.S. before, let alone taken a helicopter to a rig to conduct science at sea.
“I thought I would need a lot more experience,” she says.
But Flemings says she was ready. “One of the most exciting things I do as an educator is to just give students an opportunity to flourish,” he says. “Ultimately, Camila is a capable person.”
The cores collected during the 2017 mission were brought back to a specialized lab at the Jackson School for safe keeping and study. Since then, they have been the subject of dozens of research papers, including a special bulletin of the American Association of Petroleum Geologists.
According to Flemings, there are two major findings that have come from studying the pressure cores that guided the science on last summer’s mission.
The first finding: The 2017 cores were absolutely packed with methane hydrate. The research revealed that 79 percent to 93 percent of the pore space between the sand grains in the pressure cores was filled with the frozen gas.
However, despite the high hydrate concentration, it’s unknown where in the subsurface the methane formed and how old it is. A major focus of the science team is sampling the gas and pore water in the cores for geochemical clues that can help answer this question for Walker Ridge.
The second finding: The methane that forms the hydrate is created from microbes breaking down organic carbon buried in sediments. Flemings says this discovery came as a bit of a surprise. The oil and gas deposits in the Gulf of Mexico are the result of thermogenic processes, or the slow crushing and heating of dead organic material as it’s piled under tons of sediment over eons. Scientists presumed that the methane found in Gulf hydrate would originate from the same processes. But based on isotopic signatures from the 2017 cores, the methane isn’t produced by the gradual crushing of dead matter but as a byproduct of microbial life in action. In cores drawn from the depths, scientists are hoping to find specific microbes that call Walker Ridge home—and to investigate the role they play in both producing methane and consuming it.
The science team analyzed small samples of each conventional core that arrived on the deck of the Q4000. But these samples provided only a cursory look at the reservoir environment. The more in-depth analysis wouldn’t happen until the offshore science team and the cores returned to shore.
Nevertheless, these fleeting interactions revealed tantalizing clues about the inner workings and history of the methane hydrate reservoir at Walker Ridge.
The team discovered streaks of black iron sulfide in a core sample that might mark the past location of the layer of methane-consuming microbes. They also found a sign of where they might exist today in the form of a finger-nail-size clump of pink slime.
“[A] core can help confirm science or refute it,” says Evan Solomon, a professor and geochemist at the University of Washington. “There’s always something that we pull up in a new core that is fun or exciting.”
While at sea, scheduled science meetings kept the researchers informed on coring progress and preliminary findings. But informal discussions between colleagues were a near constant. They took place over hearty meals alongside the drill crew, while lacing up steel-toed boots, or taking a moment to watch schools of flying fish glide over the waves.
Van Der Maal says it’s these discussions that have helped her learn how science operates at the institutional level—from how grants work to finding summer research opportunities. She says she is excited to apply all she’s learned toward future projects. But there was a time not that long ago when Van Der Maal was so burned out by remote learning during the pandemic that she wasn’t sure if college was the right path for her.
During those long lockdown days, Van Der Maal and her mom would pass the time by watching videos online of Antarctic research cruises. These videos ignited a new spark of curiosity. She found herself reading about similar cruises run by UT’s Institute for Geophysics. Van Der Maal applied to UT with hopes of getting on one.
Although the Gulf of Mexico isn’t exactly the South Pole, she says the methane hydrate mission provided exactly the challenge and adventure that the Antarctic cruises inspired. She hopes that this mission is the first of many science expeditions to come.
“I’ve learned so much from everyone out here,” she says. “I can’t wait to get back offshore.”
Once the core is on deck, sampling follows a scientific protocol set in place by the science team months in advance. But when it comes to extracting the core, it’s a matter of responding to on-the-ground conditions. The swell of the sea bends the drill string, affecting drilling depth and angle. The sticky subsurface sediments often grip the coring tool and won’t let go without a fight.
Flemings and Thomas “TR” Redd, the vessel’s company man, who serves as a liaison between the science team and the drillers, spent much of their time offshore figuring out how to overcome these coring problems. The two frequently could be spotted heading back and forth between the rig office and the “doghouse,” the control room for drilling machinery just behind the drilling floor.
“Taking core is a mechanical effort,” Flemings says. “There are no automated locks or robots to put pipe into position. It’s human labor and heavy machinery.”
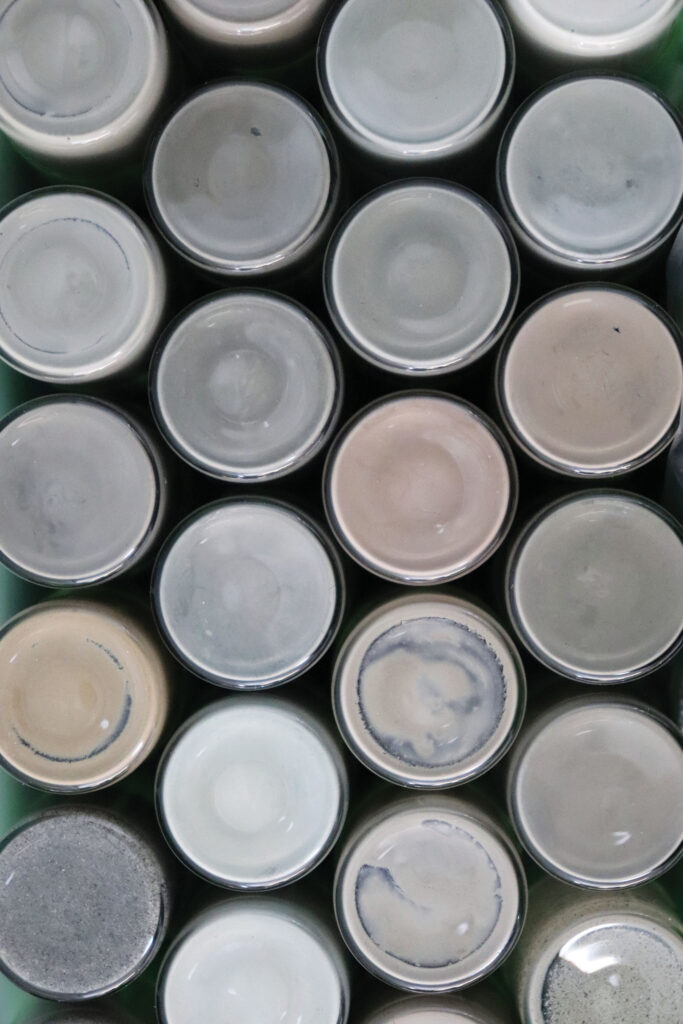
For the first week of the mission, the team overcame all the coring curve balls and hurdles. The drilling and coring progressed. But at about 500 feet down, operations suddenly came to a standstill. An essential piece of coring equipment, the fan motor on the top drive, had broken down. That same day, the vessel’s captain announced that cases of COVID-19 had sent a couple of members of the science team and the drill team into quarantine. The mission would continue. They would just have to wait out the repairs and COVID-19 cases.
In an age of digital models and automatic algorithms, this mission illustrates how pioneering science about the planet is still very much a physical process. There is no other way to get methane hydrate cores than to drill thousands of feet below the seafloor. When obstacles arise, the only way out is through.
In the midst of these issues, Flemings sent an email to the offshore science party, emphasizing the difficulty of their mission, the challenges they have overcome so far, and all they have left to do.
“Be patient … we will get there,” he wrote. “You are doing great things. I am proud to be here with you.”
A couple of days later, the cores were coming up the drill string again. The journey into the depths of the reservoir continued.
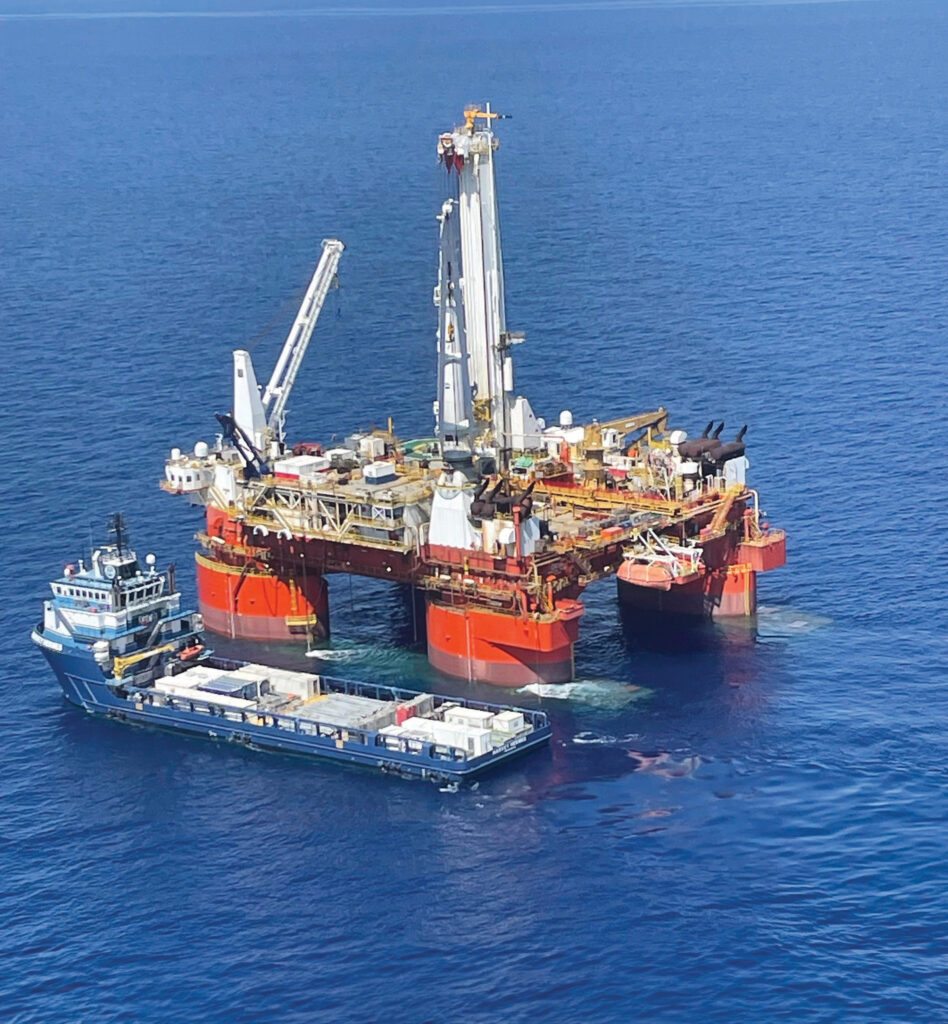
The scientists successfully retrieved a total of 44 cores from the methane hydrate reservoir: 25 pressure cores and 19 conventional cores. These are now stored at the UT Jackson School of Geosciences, where scientists will continue to study their properties and mechanics for years to come.
This story is adapted from a feature that was first published in the Jackson School’s 2023 alumni magazine, Newsletter.
CREDITS: GOM2-2 science team, USGS, courtesy of the Jackson School of Geosciences (5)
No comments
Be the first one to leave a comment.